L. Courtney Smith
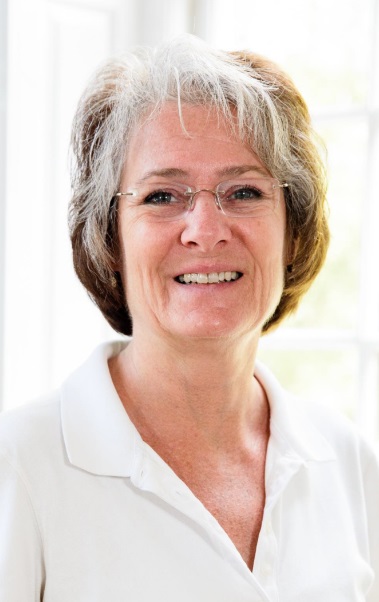
L. Courtney Smith
Professor Emerita of Biology
Cellular & Molecular Biology
Contact:
Expertise
Molecular evolution of immune systems; cell and molecular biology of immune responses in echinoderms.
The scientific question that has focused the research in the lab for many years has been ‘how do immune systems function in marine invertebrates?’ and is centered the innate immune system of the purple sea urchin, Strongylocentrotus purpuratus. Annotation of the sea urchin genome and the identification of genes encoding proteins that function in immunity demonstrated that innate immune system of this long-lived invertebrate is complex, robust, and sophisticated. Many of the immune response genes are expressed in the immune cells, or coelomocytes, that become activated in response to infection or injury. A number of immune genes are upregulated in activated coelomocytes in response to lipopolysaccharide (LPS), other pathogen associated molecular patterns, and heat-killed Vibrio diazotrophicus (a marine bacterium). These genes encode homologues of the complement cascade, lectins, a Tie receptor homologue (a putative growth factor receptor), antimicrobial peptides called SpStrongelocins, and a set of unique genes that are members of the SpTransformer (SpTrf; formerly Sp185/333) gene family that are only found in sea urchins.
The SpTrf genes are strongly upregulated in response to immune challenge. Sequences of the mRNAs and genes are unusual because optimal alignments require the insertion of large gaps that define 25-27 blocks of similar sequence called elements. The high level of sequence diversity among the messages and genes is primarily based on the presence and/or absence of elements that generate mosaics of recognizable element patterns, in addition to small indels and single nucleotide polymorphisms (SNPs). About half of the messages encode proteins with early stop codons resulting from SNPs and frameshifts that are not present in the genes. This suggests that the messages are edited, primarily prior to immune challenge when truncated proteins are produced, in which there is a preponderance of C to U substitutions in genes compared to messages. These results indicate that editing, perhaps by a cytidine deaminase, broadens the sequence diversity of the proteins.
The genes are 1.8 kb or less with two exons, of which the first encodes a leader sequence and the second encodes the mature protein. The second exon includes the mosaic pattern of elements in addition to a variety of both tandem and interspersed repeats. Computational analysis of the repeat and element sequences strongly suggests that the genes undergo frequent recombination, and molecular clock predictions suggest that the extant members of the family are evolutionarily young.
Although the sea urchin genome sequence predicts the presence of only six SpTrf genes, estimates suggest that there are 50 (+10) family members. Sequences of large genome clones called bacterial artificial chromosomes (BACs), show that the genes are tightly clustered (up to seven genes within 40 kb), and that each is surrounded by short tandem repeats (STRs). Sequencing multiple BAC inserts with SpTrf genes indicates that there are two loci of SpTrf genes with 2, 6 and 7 genes per cluster. Among the two loci, there are multiple segmental duplications that include genes and are bounded by GA STRs. The structure of the genomic regions harboring the clusters of SpTrf genes suggest significant levels of gene conversion, recombination, duplication, deletion, and general genomic instability. It is noteworthy that no pseudogenes with altered reading frames have been identified, which is very unusual for clustered genes that share sequence. These results present questions about possible mechanisms for promoting gene diversification while blocking both the formation of pseudogenes and the homogenization of entire regions from gene conversion. It is not clear whether the STRs are involved in maintaining the structure and sequence diversity of the region but their presence and positioning is intriguing, particularly the positioning of large GA STR islands in locus 2 that appear to correlate with deleted genes. The curious structure of the genomic region may be required for high rates of diversification of the SpTrf gene family, which would be beneficial to the sea urchin as an underlying mechanism to keep pace in the arms race with pathogen diversification.
The SpTrf proteins are expressed in the category of coelomocytes called phagocytes that make up about 65-75% of the cells in the coelomic (body) fluid. The SpTrf proteins are located within perinuclear vesicles of some phagocytes, and are present on the surface membrane of others. The number of SpTrf+ coelomocytes increases significantly after immune challenge, consistent with increases in gene expression, and anti-pathogen function. The proteins are secreted from the phagocytes, and opsonize marine bacteria that augments phagocytosis by the phagocytes. To date, seven recombinant (r)SpTrf proteins have been isolated, and the functions of one version (rSpTrf-E1) shows that it binds to a range of targets including Gram negative bacteria, lipopolysaccharide from E. coli, β-1,3-glucan from yeast, and phosphatidic acid that is a phospholipid without a head-group. rSpTrf-E1 does not bind to Gram positive bacteria or to peptidoglycan from Bacillus. This broad range of binding targets that have been identified for one version of the SpTrf proteins suggests that the collective functions of the variety of native proteins in sea urchins is extraordinarily effective in protecting sea urchins from the extensive range of microbes in marine systems.
The sequence diversity of immune gene families in metazoans is a consequence of the arms race between a host and its pathogens, and is directly related to the ecological niche in which it lives. The array of pathogens in contact with the host, including what the host eats and its mucosal microflora shapes the immune response and the genes that function within it over evolutionary time. Different hosts appear to have quite different means to diversify the proteins that function in pathogen detection and immune defense. Rearrangement of the immunoglobulin class of genes in higher vertebrates is a classic example of how protein sequences of antibodies and T cell receptors are diversified. The assembly of the variable lymphocyte receptors in cyclostomes is another example. However, the notion of genomic instability in a region that harbors immune genes such as the SpTrf genes is an intriguing idea for generating immune gene sequence diversity in animals that lack the molecular capabilities for gene rearrangement or assembly. There are a wide variety of how immune systems function to protect hosts from the diversity and variability of pathogens, and the sea urchin SpTrf gene family is one variation on this theme.
The SpTransformer System
Crow, RS, L Grayfer, LC Smith. 2024. Recombinant SpTransformer proteins bind to specific sites on sea urchin phagocytes and modulate SpTransformer gene expression and immune responsiveness. Frontiers in Immunology, 15:1496832. doi: 10.33389/fimmu.2024.
Crow, RS, CG Shaw, L Grayfer, LC Smith. 2024. Recombinant SpTransformer proteins are functionally diverse for binding and phagocytosis by three subtypes of sea urchin phagocytes. Frontiers in Immunology, 15:1372904.
Barela Hudgell, MA, F Momtaz, A Jafri, MA Alekseyev, LC Smith. 2024. Local genomic instability of the SpTransformer gene family in the purple sea urchin inferred from BAC insert deletions. Genes, 15(2)222.
Shaw, CG, C Pavloudi, RS Crow, JH Saw, LC Smith. 2024. Spotting disease disrupts the microbiome of infected purple sea urchins, Strongylocentrotus purpuratus. MBC Microbiology, 24:11.
Shaw, CG, C Pavloudi, MA Barela Hudgell, RS Crow, JH Saw, RA Pyron, LC Smith. 2023. Bald sea urchin disease shifts the surface microbiome on purple sea urchins in an aquarium. Pathogens and Disease, 81:ftad025.
Barela Hudgell, MA, L Grayfer, LC Smith. 2022. Coelomocyte populations in the sea urchin, Strongylocentrotus purpuratus, undergo dynamic changes in response to immune challenge. Frontiers in Immunology, 13:940852.
Barela Hudgell, MA, L Grayfer, LC Smith. 2022. A flow cytometry based approach to identify distinct coelomocyte subsets of the purple sea urchin, Strongylocentrotus purpuratus. Developmental and Comparative Immunology, 130:104352.
Barela Hudgell, MA, LC Smith. 2021. Diversity and theoretical evolutionary history of the SpTransformer genes in the sea urchin genome (pdf). Frontiers in Immunology, 12:744783.
Yakovenko, I, J Agronin, LC Smith, M Oren. 2021. Guardian of the genome: an alternative RAG/Transib co-evolution hypothesis for the origin of V(D)J recombination. Frontiers in Immunology, 12:709165.
Oren, M, B Rosental, T Hawley, G-Y Kim, J Agronin, C Reynolds, L Grayfer, LC Smith. 2019. Individual sea urchin coelomocytes undergo somatic immune gene diversification. Frontiers in Immunology, 10:1298.
Chou, H-Y, CM Lun, LC Smith. 2018. The SpTransformer proteins from the California purple sea urchin opsonize bacteria, augment phagocytosis, and retard bacterial growth. PLoS ONE 13(5):e0196890.
Lun, CM, R Samuel, SD Gillmor, A Boyd, LC Smith. 2017. SpTransformer, a recombinant Sp185/333 protein, binds to phosphatidic acid and deforms membranes. Frontiers in Immunology, 8:481.
Lun, CM, BM Bishop, LC Smith. 2017. Multitasking immune Sp185/333 protein, rSpTransformer-E1, and its recombinant fragments undergo secondary structural transformation upon binding targets. Journal of Immunology, 198(7):2957-2966.
Oren, M, MA Barela Hudgell, B D'Allura, J Agronin, A Gross, D Podini, LC Smith. 2016. Short tandem repeats, duplications, deletions, and genomic instability in a rapidly diversified immune gene family. BMC Genomics, 17:900
Lun, CM, CS Schrankel, H-Y Chou, S Sacchi, LC Smith. 2016. A recombinant Sp185/333 protein from the purple sea urchin has multitasking binding activities towards certain microbes and PAMPs. Immunobiology, 221:889-903.
Sherman, LS, CS Schrankel, KJ Brown, LC Smith. 2015. Extraordinary diversity of immune response proteins among sea urchins: nickel-isolated Sp185/333 proteins show broadvariations in size and charge. PLoS ONE, 10(19):e0138892.
Majeske, AJ, M Oren, S Sacchi, LC Smith. 2014. Single sea urchin phagocytes express messages of a single sequence from the diverse Sp185/333 gene family in response to bacterial challenge. Journal of Immunology, 195:5678-5688.
Majeske, AJ, CJ Bayne, LC Smith. 2013. Aggregation of sea urchin phagocytes is augmented in vitro by lipopolysaccharide. PLoS ONE, 8(4)e61419.
Majeske, AJ., T. Oleksyk, LC Smith. 2013. The sp185/333 immune response genes and proteins are expressed in all major organs of the adult purple sea urchin. Innate Immunity, epub.
Dheilly, NM, DA Raftos, PA Haynes & LC Smith. SV Nair. 2013. Shotgun proteomics of coelomic fluid from the purple sea urchin, Strongylocentrotus purpuratus. Developmental and Comparative Immunology, 40: 35-50.
Miller, CA, KM Buckley, RL Easley & LC Smith. 2010. An Sp185/333 gene cluster from the purple sea urchin and putative microsatellite-mediated gene diversification. BMC Genomics 11: 575. epub.
Buckley, KM, L Florea & LC Smith. 2009. A method for identifying alternative or cryptic splice sites within gene and mRNA sequences. Comparisons among sequences from vertebrates, echinoderms and other groups. BMC Genomics, 10: 318.
Dheilly, NM, SV Nair, LC Smith DA Raftos. 2009. Highly variable immune response proteins (185/333) from the sea urchin Strongylocentrotus purpuratus: proteomic analyses indicate diversity within and between individuals. Journal of Immunology, 182: 2203-2212.
Buckley, KM, DP Terwilliger, LC Smith. 2008. Sequence variations in 185/333 messages from the purple sea urchin suggest post-transcriptional modifications to increase immune diversity. Journal of Immunology, 181: 8585-8594.
Buckley, K.M., S. Munshaw, T.B. Kepler & L.C. Smith. 2008. The 185/333 gene family is a rapidly diversifying host-defense gene cluster in the purple sea urchin, Strongylocentrotus purpuratus. Journal of Molecular Biology 379: 912-928.
Brockton V., J.H. Henson, D.A. Raftos, A.J. Majeske, Y-O. Kim & L.C. Smith. 2008. Localization and diversity of 185/333 proteins from the purple sea urchin, Strongylocentrotus purpuratus (Stimpson); unexpected protein size range and expression in a new coelomocyte type. Journal of Cell Science 121: 339-348. (Cover image, Feb 1, 2008.)
Buckley, K.M. & L.C. Smith. 2007. Extraordinary diversity among members of the large gene family, 185/333, from the purple sea urchin, Strongylocentrotus purpuratus. BMC Molecular Biology 8: 68.
Terwilliger, D.P., K.M. Buckley, V. Brockton, N.J. Ritter & L.C. Smith. 2007. Distinctive expression patterns of 185/333 genes in the sea urchin; an unexpectedly diverse family of transcripts in response to LPS, β-1,3-glucan, and dsRNA. BMC Molecular Biology 8: 16.
Terwilliger D.P., K.M. Buckley, D. Mehta, P.G. Moorjani & L.C. Smith. 2006. Unexpected diversity displayed in cDNAs expressed by the immune cells of the purple sea urchin, Strongylocentrotus purpuratus. Physiological Genomics 26: 134-144. (Recommended reading by the “Faculty of 1000 Biology”, 9/2006)
Nair, S.V., H. Del Valle, P.S. Gross, D.P Terwilliger & L.C. Smith. 2005. Macroarray analysis of coelomocyte gene expression in response to LPS in the sea urchin, Strongylocentrotus purpuratus. Identification of unexpected immune diversity in an invertebrate. Physiological Genomics 22: 33-47. (Editor’s Choice, Science 310: 1871, 2005)
Reviews
Coates CJ, AF Rowley, LC Smith, MMA Whitten. 2022. Chapter 1; Host defences of invertebrates to pathogens and parasites. In: Invertebrate Pathology. AF Rowley, CJ Coates, MMA Whitten, eds. Oxford University Press. pp. 3-40.
Smith, LC, SA Boettger, M Byrne, A Hyland, DL Lipscomb, AJ Majeske, JP Rast, NW Schuh, L Song, G Tafesh, L Wang, Z Xue, Z Yu. 2022. Chapter 18; Echinoderm Diseases and Pathologies. In: Invertebrate Pathology. AF Rowley, CJ Coates, MMA Whitten, eds. Oxford University Press. pp. 505-562.
Smith, LC, M Byrne, KB Gedan, DL Lipscomb, AJ Majeske, G Tafesh-Edwards. 2022. Chapter 19; Ecological Outcomes of Echinoderm Disease, Mass Die-offs, and Pandemics. In: Invertebrate Pathology. AF Rowley, CJ Coates, MMA Whitten, eds. Oxford University Press. pp. 563-588.
Smith, LC, TA Hawley, JH Henson, AJ Majeske, M Oren, B Rosental. 2019. Methods for collection, handling, and analysis of sea urchin coelomocytes. In Methods in Cell Biology (K Foltz, A Hamdoun, eds), Elsevier. Vol 150, Part A, Chapter 16.
Smith, LC, V Arizza, MA Barela Hudgell, G Barone, AG Bodnar, KM Buckley, V Cunsolo, N Dheilly, N Franchi, SD Fugmann, R Furukawa, J Garcia-Arraras, JH Henson, T Hibino, ZH Irons, C Li, CM Lun, AJ Majeske, M Oren, P Pagliara, A Pinsino, DA Raftos, JP Rast, B Samasa, D Schillaci, CS Schrankel, L Stabili, K Stensväg, E Sutton. 2018. Echinodermata: The Complex Immune System in Echinoderms. In “Advances in Comparative Immunology”, EL Cooper, ed. Springer Publisher. Chapter 13, pp 409-501.
Smith, LC, CM Lun. 2017. The SpTransformer gene family (formerly Sp185/333) in the purple sea urchin and the functional diversity of the anti-pathogen rSpTransformer-E1 protein. Frontiers in Immunology 8:725.
LC Smith, MR Coscia. 2016. Tuning the host-pathogen relationship through evolution with a special focus on the echinoid Sp185/333 system. Invertebrate Survival Journal, 13:355-373.
Smith LC, Lun CM. 2016. Research Highlight: Invited Multitasking rSp0032 has anti-pathogen binding activities predicting flexible and effective immune responses in sea urchins mediated by the Sp185/333 system. Pathogens and Infectious Disease 2:e1394.
Oren, M, MA Barela Hudgell, P Golconda, CM Lun, LC Smith. 2016. Genomic instability and shared mechanisms for gene diversification in two distant immune gene families: the plant NBS-LRR genes and the echinoid 185/333 genes. In “The Evolution of the Immune System, Conservation and Diversification” (D Malagoli, ed.). Elsevier Inc. Academic Press, London. pp. 295-310.
Stokes, BA, S Yadav, U Shokal, LC Smith, I Eleftherianos. 2015. Bacterial and fungal pattern recognition receptors in homologous innate signaling pathways of insects and mammals. Frontiers in Microbiology 6:19.
Smith LC (2012) Innate immune complexity in the purple sea urchin: diversity of the Sp185/333 system. Frontiers in Immunology 3: 70.
Ghosh, J, CM Lun, AJ Majeske, S Sacchi, CS Schrankel, LC Smith. 2011. Invertebrate Immune Diversity. Developmental and Comparative Immunology, 35: 959-974.
Smith, L.C., J. Ghosh, K.M. Buckley, L.A. Clow, N.M. Dheilly, T. Haug, J.H. Henson, C. Li, C.M. Lun, A.J. Majeske, V. Matranga, S.V. Nair J.P. Rast, D.A. Raftos, M. Roth, S. Sacchi, C.S. Schrankel, K. Stensvåg. 2010. Echinoderm Immunity. In “Invertebrate Immunity” K. Soderhall, ed. Madame Curie Bioscience Database, Landes Biosciences, Austin TX. Advances in Experimental Medicine and Biology, 708: 260-301.
Smith, LC. 2010. Diversification of innate immune genes: lessons from the purple sea urchin. Disease Models and Mechanisms, 3: 274-279 (electronic publication).
Ghosh J, KM Buckley, SV Nair, DA Raftos, C Miller, AJ Majeske, T Hibino, JP Rast, M Roth, LC Smith. 2010. Sp185/333: A novel family of genes and proteins involved in the purple sea urchin immune response. Developmental and Comparative Immunology, (34: 235-245).
Smith, L.C., J.P. Rast, V. Brockton, D.P. Terwilliger, S.V. Nair, K.M. Buckley & A.J. Majeske. 2006. The sea urchin immune system. Invertebrate Survival Journal, 3: 25-39.
Smith, L.C. 2005. Host responses to bacteria; innate immunity in invertebrates. In The Influence of Bacterial Communities on Host Biology (M. McFall-Ngai, N. Ruby, B. Henderson, eds.). Advances in Molecular and Cellular Microbiology 10: 293-320. Cambridge University Press.
Smith, L.C., L.A. Clow & D.P. Terwilliger. 2001. The ancestral complement system in sea urchins. Immunological Reviews 180: 16-34.
Smith, L.C. 2001. The complement system in sea urchins. In Phylogenetic Perspectives on the Vertebrate Immune Systems (G. Beck, M. Sugumaran, E. Cooper, eds.). Advances in Experimental Medicine and Biology, 363-372. Kluwer Academic/Plenum Publishing Co.New York, NY.
Smith, L.C., K. Azumi & M. Nonaka. 1999. Complement systems in invertebrates. The ancient alternative and lectin pathways. Immunopharmacology, 42: 107-120.
Gross, P.S., W.Z. Al-Sharif, L.A. Clow & L.C. Smith. 1999. Echinoderm immunity and the evolution of the complement system. Developmental and Comparative Immunology, 23: 429-442.
Smith, L.C. & E.H. Davidson. 1994. The echinoderm immune system: characters shared with vertebrate immune systems, and characters arising later in deuterostome phylogeny. In: Primordial Immunity: Foundations for the Vertebrate Immune System. (G. Beck, E.L. Cooper, G.S. Habicht and J.J. Marchalonis, eds.) The New York Academy of Sciences, 712: 213-226.
Smith, L.C. & E.H. Davidson. 1992. The echinoid immune system revisited: reply. Immunology Today, 14: 93-94.
Smith, L.C. & E.H. Davidson. 1992. The echinoid immune system and the phylogenetic occurrence of immune mechanisms in deuterostomes. Immunology Today 13: 356-362.
Additional Publications On:
The Sea Urchin Genome
Rast, JP, LC Smith, M Loza-Coll, T Hibino, GW Litman. 2006. Genomic insights into the immune system of the sea urchin (PDF). Science, 314: 952-956.
Sodergren et al. (The Sea Urchin Genome Sequencing Consortium). 2006. The genome of the sea urchin Strongylocentrotus purpuratus (PDF). Science, 314: 941-952.
Hibino, T, M Loza-Coll, C Messier, AJ Majeske, AH Cohen, DP Terwilliger, KM Buckley, V Brockton, SV Nair, K Berney, SD Fugmann, MK Anderson, Z Pancer, RA Cameron, LC Smith*, JP Rast*. 2006. The immune gene repertoire encoded in the purple sea urchin genome. Developmental Biology, 300: 349-365. (*contributing authors)
The Sea Urchin Complement System
Smith LC, RS Crow, N Franchi, CS Schrankel. 2023. The echinoid complement system inferred from genome sequence searches. Developmental and Comparative Immunology, 140:104584.
Terwilliger, DP, LA Clow, PS Gross, LC Smith. 2004. Constitutive expression and alternative splicing of the SCR domains of Sp152, the sea urchin homologue of complement factor B. Implications on the evolution of the C2/Bf gene family. Immunogenetics, 56: 531-543.
Clow, LA, DA Raftos, PS Gross, LC Smith. 2004. The sea urchin homologue, SpC3, functions as an opsonin (PDF). Journal of Experimental Biology, 207: 2147-2155.
Shah, M, KM Brown, LC Smith. 2003. The gene encoding the sea urchin complement protein, SpC3, is expressed in embryos and can be upregulated by bacteria (PDF). Developmental and Comparative Immunology, 27: 529-538.
Smith, LC. 2002. Thioester function is conserved in SpC3, the sea urchin homologue of the complement component C3 (PDF). Developmental and Comparative Immunology, 26: 603-614.
Clow, LA, PS Gross, C-S Shih & LC Smith. 2000. Expression of SpC3, the sea urchin complement component, in response to lipopolysaccharide (PDF). Immunogenetics, 51: 1043-1044.
Gross, PS, LA Clow, LC Smith. 2000. SpC3, the complement homologue from the purple sea urchin, Strongylocentrotus purpuratus, is expressed in two subpopulations of the phagocytic coelomocytes (PDF). Immunogenetics, 51: 1021-1033.
Smith, LC, C-S Shih, SG Dachenhausen. 1998. Coelomocytes specifically express SpBf, a homologue of factor B, the second component in the sea urchin complement system (PDF). Journal of Immunology, 161: 6784-6793.
Al-Sharif, WZ, JO Sunyer, JD Lambris, LC Smith. 1998. A homologue of the complement component C3 is specifically expressed in sea urchin coelomocytes (PDF). Journal of Immunology, 160: 2983-2997.
Sea Urchin Gene Expression and Other Topics
Golconda P, Buckley KM, Reynolds CR, Romanello JP, Smith, LC. 2019. The axial organ and the pharynx are sites of hematopoiesis in the sea urchin. Frontiers in Immunology 10:870.
Stevens, M.E., J. Dhillon, C. Miller, C. Messier-Solek, J.P. Rast, A.J. Majeske, D. Zuelke, L.C. Smith. 2010. SpTie1/2 from the sea urchin Strongylocentrotus purpuratus, is an orthologue of vertebrate Tie1 and Tie2 and is expressed in coelomocytes, axial organ and embryos (PDF). Developmental and Comparative Immunology, 34(8):884-895.
Li, C., H-M. Blencke, L.C. Smith, M.T. Karp, K. Stensvag. 2009. Two recombinant peptides, SpStrongylocins 1 and 2, from Strongylocentrotus purpuratus, show antimicrobial activity against Gram-positive and Gram-negative bacteria (PDF). Developmental and Comparative Immunology, 34(3): 286-292.
Multerer, KA, LC Smith. 2004. Two cDNAs from the purple sea urchin, Strongylocentrotus purpuratus, encoding mosaic proteins with domains found in factor H, factor I, and complement components C6 and C7 (PDF). Immunogenetics, 56: 89-106.
Smith, LC, L Chang, RJ Britten, EH Davidson. 1996. Sea urchin genes expressed in activated coelomocytes are identified by expressed sequence tags (ESTs). Complement homologues and other putative immune response genes suggest immune system homology within the deuterostomes (PDF). Journal of Immunology, 156: 593-602.
Smith, LC, RJ Britten, EH Davidson. 1995. Lipopolysaccharide activates the sea urchin immune system (PDF). Developmental and Comparative Immunology, 19: 217-224.
Smith, LC, MG Harrington, RJ Britten, EH Davidson. 1994. The sea urchin profilin gene is expressed in mesenchyme cells during gastrulation (PDF). Developmental Biology, 164: 463-474.
Cameron, R.A., L.C. Smith, R.J. Britten & E.H. Davidson. 1994. Ligand-dependent stimulation of introduced mammalian brain receptors alters spicule symmetry and other morphogenetic events in sea urchin embryos. Mechanisms of Development 45: 31-47.
Smith, LC, RJ Britten, EH Davidson. 1992. SpCoel1, a sea urchin profilin gene expressed specifically in coelomocytes in response to injury (PDF). Molecular Biology of the Cell, 3: 403-414
Immune Responses in Marine Sponges
Smith, LC. 2024. The marine sponge, Hymeniacidon sinapium, displays allorecognition of siblings during post-larval settling and metamorphosis to juveniles. Developmental and Comparative Immunology, 157:105179
Smith, L.C. & W.H. Hildemann. 1990. Cellular morphology of Callyspongia diffusa (Porifera: Demospongia) (PDF). In: New Perspectives in Sponge Biology. (K. Ruetzler & W. Hartman, eds.) Smithsonian Institution Press, Washington, D.C., pp. 135-143.
Smith, L.C. 1988. The role of mesohyl cells in sponge allograft rejection (PDF). In: Invertebrate Historecognition (R.K. Grosberg, D. Hedgecock & K. Nelson, eds.). Plenum Publishing Co., pp. 15-30.
Smith, L.C. & W.H. Hildemann. 1986. Allogeneic cell interactions during graft rejection in Callyspongia diffusa (Porifera, Demospongia); a study with monoclonal antibodies (PDF). Proceedings of the Royal Society of London B 266: 465-477.
Smith, L.C. & W.H. Hildemann. 1986. Allograft rejection, autograft fusion and inflammatory responses to injury in Callyspongia diffusa (Porifera, Demospongia) (PDF). Proceedings of the Royal Society of London B 266: 445-464.
Smith, L.C. & W.H. Hildemann. 1984. Alloimmune memory is absent in Hymeniacidon sinapium, a marine sponge (PDF). Journal of Immunology 135: 2351-2355.
B.A., Drake University, 1974
M.S., University of Minnesota, 1976
Ph.D., University of California, Los Angeles, 1985, with William Hildemann
Post-Doctoral Work: California Institute of Technology with Eric Davidson
BISC 3212 - Immunology